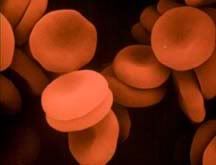
Oxygen, O2, scavenger of electrons, oxidizer of molecules. Given a spark, it will react with molecules in a combustion explosion, given the time it will eat away iron producing rust, if let loose in the body it will wreak havoc, leaching the electrons strait from your tissues. For as good a reputation as it has with us, oxygen is down right murderous. So why do we need? And how do we manage it? If you ask me, it just sounds like a bad idea to begin with. But really, the use of oxygen in metabolism had very clever beginnings…Oxygen wasn’t so popular at first, let me tell ya. The first organisms arose about 3.5 billion years ago when free oxygen was a rarity. They were anaerobic cells, and just as their name indicates, they had no use for oxygen. Originally, the process of glycolysis was used to break down organic compounds found in their environment. When the abundance of free organic compounds began to dwindle, photosynthetic organisms that could fix carbon dioxide (CO2) in the air to make their own organic compounds arose. A side effect of the ingenious design of photosynthesis just happened to be molecular oxygen (O2). There was very little O2 in the air at that time, except what they produced, and like us they tended to just let their waste build up (being unicellular, they didn’t exactly have the most creative waste management!). By about 2.5 billion years ago, the levels of oxygen began to poison these anaerobes. Dieing away in their own waste, these organisms seemed doomed…until a new organism hit the scene. One microbe’s trash is another microbe’s treasure, as they say, and an organism arose that took the idea behind photosynthesis, and practically put it in reverse. These organisms would utilize oxygen, and produce CO2 as a byproduct. Many of you have heard this story before, about how our ancestral mitochondrions saved the day and the entire earth from an overly oxidizing atmosphere. But the story goes deeper still.
About 1.2 billion years ago the first multicellular organisms appeared. It was easy at first, getting oxygen to a limited number of cells took nothing more than simple diffusion. As organisms became larger and more complex, around 550 million years ago, a little more effort had to go into getting that oxygen where it was needed. Gastrovascular cavities were a simple way to go about it.
What about a creature like us though? A large creature with a diverse variety of tissues, each with their own special functions, from the tip of the head to the bottom of its toes? Clearly, diffusion is not going to cut it in an organism like us. Oxygen must be carried where it is needed, and CO2 taken away. This requires an efficient system of transport—a cardiovascular superhighway.
Oxygen transport is no small task. As we know, O2 is dangerous stuff; our blood cells might as well be carrying bombs. So how do they do it, these little erythrocytes (the scientific term for red blood cells, I’d like to use it in this article because it’s a very pretty word)? Like super secret agents, they package O2 very carefully in their specialized hemoglobin proteins.
Hemoglobin is a protein with four subunits. It’s not all amino acids; each subunit also contains a prosthetic group, a non amino acid component that is essential to its function. This group, called the heme group, has a little something that oxygen loves very much: iron. Normally oxygen’s relationship with iron is, well, a little abusive. Oxygen takes electrons from iron atoms and leaves iron in an oxidized state, forming rust. The heme group takes precautions against such abuse. The iron is secured all around by a nitrogen ring that holds it steady with a distal histidine guarding its back. Histidine is one of the amino acids comprising hemoglobin and plays two necessary roles. For one, it hydrogen bonds the other end of the O2 molecule, keeping it in place. The other role is dealing with carbon monoxide. Carbon monoxide (CO) also has a love of iron, an affinity that is thousands of times greater than O2s. Luckily, the distal histidine gets in the way of what could be a beautiful relationship between the iron and CO. The distal histidine presses down into the plane where the CO would normally bind, forcing a bent conformation. CO is linear and unhappy in such a state, but O2, when bound to the iron, has no problem being bent and is comfortable at an angle.
So the hemoglobin has packaged its oxygen. Very good, but it still must release this precious load to tissues that need it. How does it know when it’s at just the right place? Several things cue in the hemoglobin molecule. Active tissues are more acidic, with a higher concentration of CO2 and a lower concentration of O2. They also create the molecule 2,3-Bisphosphoglycerate (2,3 BPG) while breaking down glucose. Together, all of these molecules help to produce a conformational change in the hemoglobin protein complex. It releases it's oxygen and is now in the deoxygenated state and ready to pick up CO2. Check here to see the way hemoglobin moves to bind and release oxygen: http://en.wikipedia.org/wiki/Image:Hemoglobin_t-r_state_ani.gif
I hope you’ve enjoyed this article on oxygen and erythrocytes! It is in part an attempt to get you geared up for my next article though—I’d like to tell you all about sickle cell anemia, why it persists in some countries, and what causes those erythrocytes to sickle. Stay tuned for that this Wednesday.
Sources:
U of I's own Dr. Cole
Campbell. Biology, 4th Edition. 1996
Purves, Sadava, Orians, Heller. Life, the Science of Biology, 6th Edition. 2000
Images:
Cartoon: http://www.snow.edu/~kevins
Erythrocytes: encarta.msn.com
Heme and hemoglobin: wikipedia
0 Comments:
Post a Comment
<< Home